Gregor Schiwietz
Auger-, convoy- and delta-electron spectroscopy
Technical:
The ion beam of a few MeV per nucleon is delivered by the ISL cyclotron. Foil strippers allow to increase the charge state and for typical experiments on ion-solid interactions the mean equilibrium charge state is magnetically selected prior to entering the scattering chamber.
Experimental setup for high-energy ion/solid interactions
It consists of:
- A mu-metal shielded scattering chamber (residual gas pressure below 1 micro torr and residual magnetic field < < 1% of earths magnetic field)
- A target wobbler (brown) to carry and control the position of the solid-state targets(a measurement of the target current is possible).
- A gas cell (black) for the purpose of comparison with atomic targets.
- Electrostatic electron spectrometers (blue).
- Surface-barrier detectors (green) for the measurement of projectile energy losses and in-situ determination of the target composition.
- A Faraday cup for the beam-current normalization.
- An electron gun (not shown in the figure) for test purposes as well as for the comparison electron vs. ion irradiation.
- A quadrupole mass-spectrometer (not shown in the figure) for the investigation of ion-induced desorption.
Selected references on ion/solid interactions:
- G.Schiwietz, D.Schneider and J.Tanis; "Formation of Rydberg States in Fast Ions Penetrating Thin Carbon-Foil and Gas Targets" , Phys. Rev. Lett. 59, 1561 (1987)
- G.Schiwietz, D.Schneider, J.P.Biersack, N.Stolterfoht, D.Fink, A.Mattis, B.Skogvall, H.Altevogt, V.Montemayor, and U.Stettner; "Cascade-Induced Asymmetry in Auger-Electron Emission Following Fast Ion-Solid Interactions" , Phys.Rev.Lett. 61, 2677 (1988)
- G.Schiwietz, J.P.Biersack, D.Schneider, N.Stolterfoht, D.Fink, V.Montemayor, and B.Skogvall; "Investigation of Delta-Electron Production in Collisions of Highly Charged Fast Ne Projectiles with Carbon-Foil Targets" , Phys.Rev. B41, 6262-71 (1990)
- G.Schiwietz, P.L.Grande, B.Skogvall, J.P.Biersack, R.Köhrbrück, K.Sommer, A.Schmoldt, P.Goppelt, I.Kadar, S.Ricz, and U.Stettner; "Influence of Nuclear Track Potentials in Insulators on the Emission of Target Auger Electrons" , Phys.Rev.Lett. 69, 628 (1992)
- G.Schiwietz and G.Xiao; "Electron Ejection from Solids Induced by Fast Highly-Charged Ions" , NIM B107, 113-127 (1996)
- G.Xiao, G.Schiwietz, P.L.Grande, N.Stolterfoht, A.Schmoldt, M.Grether, R.Köhrbrück, A.Spieler and U.Stettner; "Indications of Nuclear-Track-Guided Electrons Induced by Heavy Ions in Insulators" , Phys. Rev. Lett. 79, 1821-1824 (1997)
- G. Schiwietz, G. Xiao, P.L. Grande, E. Luderer, R. Pazirandeh, U. Stettner; "An Experimental Determination of Electron Temperatures in the Center of Nuclear Tracks in Amorphous Carbon", Nucl. Instr. Meth. B146 , 131-136 (1998)
- G. Schiwietz, G. Xiao, P.L. Grande, E. Luderer, R. Pazirandeh, U. Stettner; "Determination of the electron temperature in the thermal spike of amorphous carbon", Europhys. Lett. 47, 384-390 (1999)
- G. Schiwietz, G. Xiao, E. Luderer, and P.L. Grande; "Auger Electrons from Ion Tracks", Nucl. Instr. Meth. B164-165, 353-364 (2000)
- G. Schiwietz, E. Luderer, G. Xiao and P.L. Grande; "Energy Dissipation of Fast Heavy Ions in Matter", Nucl. Instr. Meth. B175-177, 1-11 (2001)
- G. Schiwietz, K. Czerski, M. Roth, F. Staufenbiel, E. Luderer, P.L. Grande; "Si-Auger electrons from the center of nuclear track", Nucl. Instr. Meth. B193, 705-712 (2002)
- G. Schiwietz, M. Roth, K. Czerski, F. Staufenbiel, and P.L. Grande; "Femtosecond Dynamics - Snapshots of the Early Ion-Track Evolution", Nucl. Instr. Meth<//span>. B225, 4-26 (2004) and Nucl. Instr. Meth. B226 (2004) 683–704
- F. Staufenbiel, G. Schiwietz, K. Czerski, M. Roth, and P.L. Grande; "Electronic energy-density effects in ion tracks of metals", Nucl. Instr. Meth. B230, 426–430 (2005)
- G. Schiwietz, M. Roth, K. Czerski, F. Staufenbiel, and P.L. Grande; "Indications for enhanced Auger-electron absorption in a hot electron gas", Phys.Rev.Lett. 99, 197602 (2007)
- G. Schiwietz, K. Czerski, R. Hellhammer, M. Roth, F. Staufenbiel, R.C. Fadanelli, and P.L. Grande; "Search for short-time phase effects in the electronic damage evolution - a case study with silicon", Nucl. Instr. Meth. B266, 1287–1293 (2008)
- P.L. Grande, L.C.C.M. Nagamine, J. Morais, M.C.M. Alves, G. Schiwietz, M. Roth, B. Schattat, and E. Baggio-Saitovitch; "High-energy ion beam irradiation of Co/NiFe/Co/Cu multilayers: Effects on the structural, transport and magnetic properties", Thin Solid Films 516 (8), 2087-2093 (2008)
- R.M. Papaléo, M.R. Silva, R. Leal, P.L. Grande, M. Roth, B. Schattat, and G. Schiwietz; "Direct evidence for projectile charge-state dependent crater formation due to fast ions", Phys.Rev.Lett. 101, 167601 (2008)
Theoretical results on high-energy ion/solid interactions
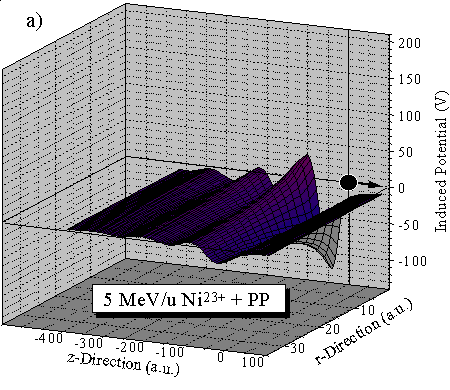
Figure 2a):
Induced (wake) potential for 5 MeV/u Ni23+ ions penetrating polypropylene (PP). The projectiles move with a speed of 14.1 a.u. in the positive z-direction. Calculations where performed in the linear-response theory for ahomogeneous electron gas using a generalized plasmon-pole dielectric function (see Ritchie and Echenique et al.). Thus, the electronic motionis influenced by the projectile potential (in a perturbative treatment) as well as by the collective electron-electron interaction. Interactions with the screened target-core potentials have not been accounted for.
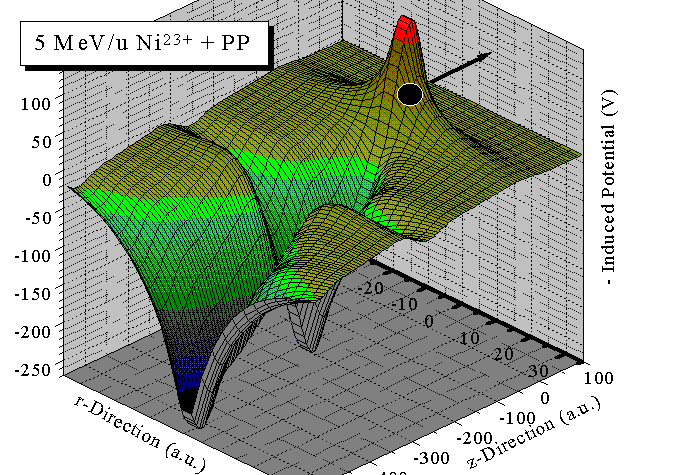
Figure 2b):
Nuclear-track potential for 5 MeV/u Ni23+ ions penetrating polypropylene. The projectiles move with a speed of 14.1 a.u. in the positive z-direction. Calculations where performed using anon-perturbative classical-trajectory Monte Carlo code forthe time dependent electron density. Thus, the electronic motionis influenced by the projectile potential as well as by the screened target-core potentials. Furthermore, in this model free electrons slow down continuously in the medium until they stop. Collective effects and electron recombination have not been accounted for.
It is seen that Fig. 2a displays oscillations (due to the averageelectron-electron interaction). The projectile attracts the electrons (first minimum of the induced potential directly behind the projectile) and the electrons repel each other (this gives rise to the oscillations further behind the projectile). For conduction-band electrons in a metal, where the influence of the target cores can be neglected, the linear-response theory is expected to be accurate as long as the projectile is fast and has a small nuclear charge. Contrary, for highly charged ions in insulators the linear-response theory is expected to fail. If furthermore electron recombination is slow, the track modelof Fig. 2b is expected to be more accurate. It neglects collective effectsand it underestimates the long-range interaction between projectile and electrons, but these effects are of minor importance for heavy projectiles. In fact, nuclear-trackpotentials, consistent with Fig. 2b, have been found experimentally for polypropylene targets (see refs. d, f and g).